The Influence of Soot on Evacuation Characteristics of Diesel Exhaust Particles: An Inhalational Toxicology Study
Article information
Abstract
Despite being a major combustion product during fires, soot's short-term inhalation effects are considered negligible, leading to its exclusion from the FED model. However, long-term soot exposure, prevalent due to air pollution, is linked to lung inflammation and other health concerns. Interestingly, the impact of chronic soot inhalation on motor ability remains largely unexplored, affecting not only occupational groups but also the broader population. This pilot study aimed to address this gap by investigating the toxicity of continuous soot inhalation through diesel exhaust particles (DEPs) in laboratory mice. We employed the open field maze text to assess changes in motor behavior following DEP exposure. Our results suggest a correlation between increased DEP inhalation, elevated lung cell inflammation, and impaired motor function in mice. Further research is warranted to quantitatively evaluate the impact of soot on motor ability.
1. Introduction
Hydrogen cyanide, carbon monoxide, and carbon dioxide are the main toxic combustion products in a fire, and their inhalation is fatal to evacuees[1]. Commonly applied evacuation models include FDS+EVAC[2], EXODUS[3], and PATHFINDER[4], while PURSER's FED model[5] specifically reflects the toxicity effect of fire.
Soot, though a major combustion product, is disregarded in the FED model due to its negligible short-term effects upon inhalation. However, long-term soot inhalation can trigger lung cell inflammation and even lung tumors[6]. Seelig et al. verified that long-term inhalation of coal combustion soot can cause lung tumors[7], and Kotin et al. confirmed carcinoma in mice exposed to soot from gasoline exhaust[8,9]. Park and Han observed that firefighters, facing chronic exposure, develop inflammatory responses in various organs leading to asthma, respiratory diseases, and cardiovascular issues[6].
With the recent intensification of air pollution, not only are workers in relevant fields exposed to increased risk of inhaling soot, diesel exhaust particles (DEPs), and particulate matter, but also the general population faces greater danger. DEP, a major air pollutant, is a complex mixture of organic compounds predominantly composed of carbon crystals[10]. This similarity in composition allows us to hypothesize that soot and DEPS may be functionally identical[11]. In the fields of medicine and nutrition, soot and DEPs are often treated as equivalent substances, prompting active research into their effects on the human body.
J. Kagawa classified soot as a carcinogen, and linked short-term inhalation to vertigo and nausea. Long-term exposure has also been associated with cardiopulmonary diseases[12]. Similarly, the U.S. National Institute for Occupational Safety and Health reported that high-concentration DEP exposure in work environments significantly increases the risk of lung cancer, suggesting a potential role in activating carcinogenesis[13].
Despite numerous studies exploring the medical implications of soot inhalation, their impact on motor function remains largely unexplored. Therefore, we conducted an experimental study to investigate the effects of long-term soot inhalation on motor ability characteristics. Given the ethical and practical limitations of inhalational toxicology studies on humans, we employed an animal experiment using laboratory mice. DEPs were chosen as the inhalational test substance due to their similarities to soot. Furthermore, an open field maze (OFM) test was utilized to assess the impact of DEP inhalation on the psychological factors influencing motor ability in mice.
2. Research Method
2.1 DEP inhalation toxicity test
To analyze the changes in mice's motor characteristics upon soot inhalation, we performed an inhalation toxicity test using DEP. We simulated DEP adhesion in the lungs by directly administering a diluted PEP solution in physiological saline to the mice's airways.
Table 1 outlines the experimental conditions based on DEP dosage. We prepared diluted DEP solutions at 0 mg/kg, 5 mg/kg, and 15 mg/kg to analyze how behavioral characteristics change with DEP exposure levels. We defined the groups receiving these solutions as CTRL, DEPL, and DEPH, respectively. Each group comprised eight mice, administered with their assigned DEP solution for seven consecutive days. After inhalation, we used the OFM to examine characteristic changes in mouse motor ability. Finally, the mice were sacrificed for post-mortem lung cell analysis.
2.2 OFM
Commonly used experimental models for analyzing motor changes in laboratory animals include the elevated plus maze[14], Y maze[15], forced swimming test[16], and OFM[17]. Among these, the OFM is particularly favored for assessing anxiety-related motor ability changes and exploring animal behavior in response to diverse stimuli[18]. It helps predict correlations between mouse motor and psychological characteristics based on their preference for dark, secluded areas.
We hypothesized that DEP-induced ailments would ultimately affect motor function through altered psychological states. Therefore, the OFM was chosen in this study to analyze the changes in motor characteristics of mice following DEP inhalation.
Figure 1 depicts the OFM experiment setup. Mice were placed in an open field (0.42 × 0.42 × 0.42 m3), and their subsequent behavior was analyzed. The OFM allows the prediction of psychological characteristics (anxiety, etc.) based on the areas occupied by the mice, enabling us to determine the correlation between the predicted anxiety levels and motor measures like distance and speed.
3. Experiment Results
3.1 Cell contents in the bronchoalveolar lavage fluid (BALF)
Given our hypothesis that DEP-induced ailments would affect motor function in mice, we performed lung tissue biopsies to analyze the inflammatory response characteristics following DEP inhalation.
Figure 2 shows lung tissue images across the experimental conditions. The blue arrow highlights an increasing level of DEP adhesion in lung cells as the DEP dosage rises. The red arrow points to the concomitant rise in lung cell inflammation with increasing DEP adhesion. However, we acknowledge that visual assessment of lung cell images alone cannot definitively quantify the level of inflammation. Therefore, we employed a BALF test. This involved injecting physiological saline into the lungs to quantitatively analyze the inflammatory cell content in the alveoli[20].
Figure 3 presents the estimated inflammatory cell counts from the BALF analysis. Panel (a) shows the total cell number, while panels (b), (c), (d), and (e) detail the specific counts for macrophages, neutrophils, eosinophils, and lymphocytes, respectively. Analyzing these graphs provides important insights into the cell types involved in the inflammatory response.

Inflammatory cell profile in the bronchoalveolar lavage fluid: (a) total cell number, (b) macrophages, (c) neutrophils, (d) eosinophils, and (e) lymphocytes.
As Figures 3(b) and 3(c) demonstrate, both macrophage and neutrophil count significantly increased with increasing DEP inhalation levels. This observation aligns well with the known functions of these cell types - macrophages participate in respiratory defense, while neutrophils suppress foreign substances.
Figures 3(d) and 3(e) reveal negligible changes in eosinophil and lymphocyte counts compared to the marked increases in macrophages and neutrophils. This suggests that the induced inflammation is not primarily driven by parasitic infection, allergic reactions, or bacterial/viral causes, which typically involve substantial activation of eosinophils and lymphocytes, respectively. Therefore, the observed lung cell inflammation is most likely a direct consequence of DEP inhalation, with higher exposure levels correlating with a more pronounced inflammatory response.
3.2 Open field test result
Figure 4 illustrates our method of predicting the psychological characteristics of mice in an OFM based on their movement patterns. The OFM is divided into (a) a center area (a relatively open and brightly lit space) and (b) a border area (a darker and more enclosed zone along the walls of the maze). We leverage the natural tendency of mice to prefer secluded areas as a basis for predicting their psychological state. Mice with a normal, calm state are expected to spend most of their time in the border area. Conversely, as anxiety levels increase due to external factors, mice will exhibit increased frequency of movements, prolonged time spent in the center area, and greater distance traveled in the center area. By analyzing these parameters derived from their movement patterns, we can predict the level of anxiety induced by the external factor.
Figure 5 presents the cumulative distances traveled by mice in the OFM, broken down by area: (a) total distance, (b) distance in the border area, and (c) distance in the center area.
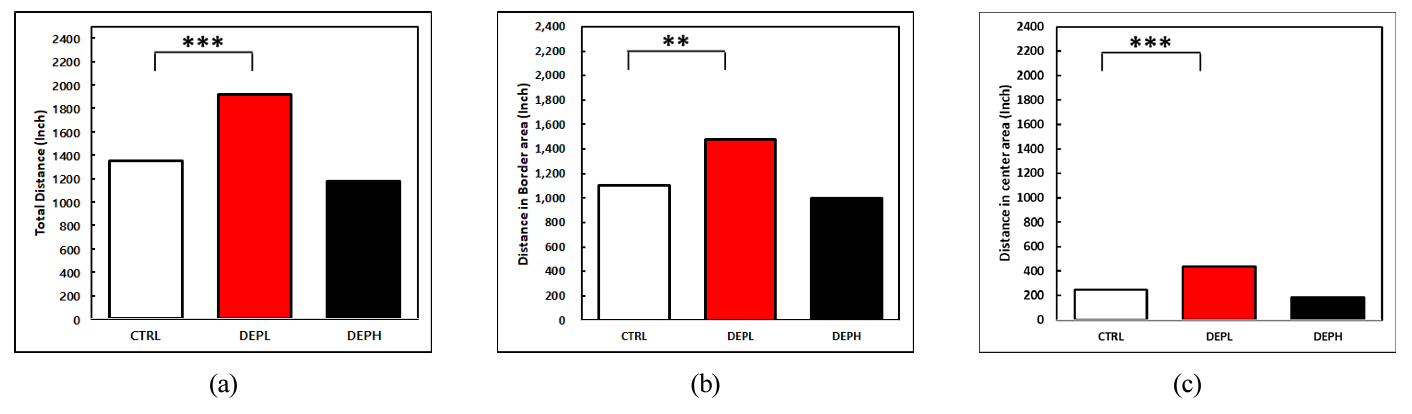
Distance traveled by mice (a) total distance, (b) distance in border area, (c) distance in center area. The one-way ANOVA was followed by Tukey's post-hoc test; * p < 0.05; ** p < 0.01; *** p < 0.001.
The data reveals a complex relationship between DEP inhalation, anxiety, and motor ability. Comparing the DEPL group (low DEP exposure) to the CTRL group (no DEP), we see significant increases in both total distance traveled (a) and the distance specifically within the center area (c). This suggests that a moderate level of DEP-induced lung inflammation (as shown in Figure 3) might initially elevate anxiety levels in mice, leading to greater movement and exploration.
However, the DEPH group (high DEP exposure) paints a different picture. Here, the total distance traveled (a) significantly decreased to the CTRL group. This implies that severe lung inflammation from high DEP exposure ultimately impairs motor function and reduces overall activity. While Figure 3 again shows heightened inflammation in this group, the stark contrast in behavior highlights the complex interplay between inflammation and its impact on motor ability. It is crucial to note that further statistical analysis is required to definitively confirm the correlation between changes in motor ability and anxiety levels arising from DEP-induced lung inflammation.
Figure 6 depicts the mean speed of mice in the OFM subdivided into: (a) total mean speed, (b) mean speed in the border area, and (c) mean speed in the center area. These speeds were calculated by dividing the distance traveled in each area by the corresponding time spent there.

Mean speed (a) the total mean speed, (b) the mean speed in the border area, and (c) the mean speed in the center area. The one-way ANOVA was followed by Tukey's post-hoc test; ***p < 0.001.
The data reveals notable parallels between mean speed trends (Figure 6) and distance trends (Figure 5). Anxiety-induced increases in mean speed are observed in both total and border areas, aligning with the elevated distances observed in Figure 5. This suggests that moderate lung inflammation might initially drive heightened activity and faster movements. Mean speed decreases in both total and border areas, mirroring the reductions in distance. This supports the notion that severe lung inflammation ultimately impairs motor function and slows movement.
However, an intriguing divergence emerges in the center area. DEPL mice traveled significantly farther in the center area compared to CTRL mice. This difference in distance was not accompanied by a significant change in mean speed. the change in the mean speed showed no significance. This discrepancy suggests that DEPL mice might have exhibited increased freezing behavior within the center area, despite covering more ground.
4. Conclusion
This study employed an inhalation toxicity test using DEP to analyze changes in mice's motor characteristics with respect to soot inhalation. Post-mortem examinations further confirmed that DEP inhalation induces lung cell inflammation. Moreover, an OFM test explored how behavioral characteristics change with DEP exposure. Based on the results, the following conclusions were drawn.
1. The BALF test revealed a dose-dependent increase in total inflammatory cell content. Analysis of the specific functions of each cell type implicated inflammatory response to DEP inhalation rather than parasitic infection, allergic reaction, or bacterial/viral causes.
2. The OFM test results indicated that DEP inhalation influenced both distance traveled and mean speed. While the precise correlation between these changes and DEP levels remained unverified, the study demonstrated a significant impact of anxiety triggered by cell inflammation on motor ability.
3. In summary, long-term soot inhalation was shown to trigger bronchial inflammation, ultimately impacting motor function. This underscores the need for future studies to quantitatively analyze each contributing factor when assessing the effects of soot inhalation on motor ability.
Notes
Author Contributions
Writing original draft preparation, Writing review and editing, B.W. SO; Data curation, investigation, S.Y. JEONG; Supervision, Project administration, S. BAE; Writing review and editing, H.J. JEONG. All the authors have read and agreed to the published version of the manuscript.
Conflicts of Interest
The authors declare no conflict of interest.
Acknowledgements
This research was supported by "Regional Innovation Strategy (RIS)" through the National Research Foundation of Korea(NRF) funded by the Ministry of Education(MOE) (2021RIS-002).